About us / info / Regulations
Are you really protected against X-rays at your clinic ?
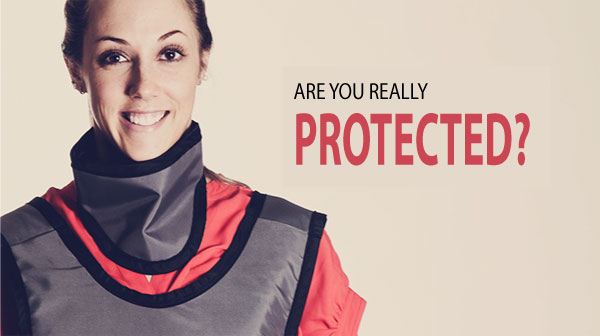
This is a question that must be asked when we are using an
X-ray machine. Several elements of your protective clothing should be checked to ensure that the clinic staff is well protected when taking X-ray of a patient. According to Health Canada, a law regulates radiation protection for protective clothing in a radiology ward1.
Protection clothing required when taking X-rays are the apron, gloves or mittens, and the thyroid gland guard. These three elements are required to ensure maximum security.
Why do I need to protect these regions ?
When taking x-rays, only parts of the body most at risk of exposure are protected. The thyroid gland, lungs and heart are at greater risk of getting cancer.
Thickness :
Protective clothing must ensure lead thickness equivalent of at least 0.5 mm for a voltage of 150 kVp.
Labeling :
Lead thickness of the material must be permanently and legibly marked on each garment, at all times. Otherwise, the product is considered non-regulatory.
Storage :
All protection clothing must be stored in accordance with manufacturer's recommendations. To prevent lead damage, it is recommended hanging clothes on a holder designed for this purpose. If heavy objects are placed on the protective clothing, there is a risk that the lead might crack.
Check your protective clothing :
An x-ray of each garment is highly recommended once a year or when a defect is suspected. This will ensure that the lead is in perfect condition, which means without any cracks. If this is not the case, the radiation can reach your organs.
1* Protective Clothing Regulation 6.2 :
Protective aprons, gloves and the thyroid gland guard used for radiological examinations in veterinary medicine must provide lead thickness equivalent of at least 0.5 mm for a voltage of 150 kVp. The lead equivalent thickness of the material used must be permanently indicated and legibly marked on the protective devices. Gloves should ensure full protection of the hand, fingers and including the wrist.
Protective aprons, gloves and the thyroid gland guard must be stored and maintained in accordance with manufacturer's recommendations. It is also advisable to check by an x-ray all clothing and protective devices once a year or when a damage is suspected.
Source:
www.hc-sc.gc.ca
Analysis of Univest-X Apron Lead-Equivalent Thickness
I.A. Cunningham, PhD, FCCPM, FAAPM
Professor, The University of Western Ontario
March 23, 2006
Executive Summary
The protective value of Univest-X apron lead for radiation protection in diagnostic radiology was measured using x-ray spectra from 60 to 150 kV. The exposure protection factor was compared with the protection offered by pure lead and expressed in terms of an equivalent lead thickness.
Two samples were received from Univest-X Inc for analysis.
Sample #1 consisted of two layers with a total nominal lead-equivalent thickness of 0.5 mm.
Sample #2 consisted of nominal lead-equivalent thickness of 0.25 mm in one layer.
Results are summarized in the following table. In all cases, the measured exposure protection factor was consistent with the stated lead-equivalent thickness within the accuracy of the measurement. It is concluded that sample #1 has a lead-equivalent
thickness of 0.5 mm and sample #2 has a lead-equivalent thickness of 0.25 mm as claimed by the manufacturer over the energy range of 60 to 150 kV.
Stated Lead-Equivalent Measured Lead-Equivlent
Thickness (mm) Thickness (mm)
Sample #1 (two layers) 0.5 0.5
Sample #2 (one layers) 0.25 0.25
Introduction
Federal and provincial legislations impose minimum requirements on the effectiveness of x-ray protective devices in terms of a thickness of lead in mm. Some protective aprons and other garments are made of materials other than lead. This report summarizes a calculation in which the protective value of non-lead materials is determined and expressed as a “lead-equivalent” thickness. With this testing, it is known that a material specified as having a certain Pb-equivalent thickness truly provides the same protection as the stated thickness of pure lead.
The protection factor, P, is the ratio of Xo, exposure (in mR) received without the protective device, to X, exposure with the protective device. Thus, the exposure received by someone wearing the protection, X, is given by X = Xo / P.
Figure 1 shows a theoretical calculation of the protection factor offered by 0.5 mm of pure lead as a function of x-ray energy. Because of this strong energy dependence, the lead-equivalent thickness of a material can only be determined for an x-ray spectrum of known shape.
Protection Factor of 0.5 mm of Lead
Protection
Factor
100
10
1
0 50 100 150
X-Ray Energy (keV)
Figure 1. Protection factor of 0.5 mm of pure lead as a function of x-ray energy.
Methods
Evaluation of lead-equivalent thickness is completed with the following steps.
1. Characterize spectra covering a range of energies in the diagnostic spectrum in terms of kV and half-value layer.
2. Measure the protection factor of each sample with each spectrum.
3. Determine lead-equivalent thickness of each sample with each spectrum based on the measured protection factor.
4. Confirm that scatter from the samples is comparable with scatter from pure lead of the same thickness.
5. Determine the lead-equivalent thickness of each sample plus a piece of pure lead of known thickness. The lead result is used to confirm accuracy of the analysis by demonstrating that the lead-equivalent thickness is equal to the actual thickness.
Samples were examined as described in the following table.
Stated Lead Equivalence
Sample #1 Two layers 0.5 mm
Sample #2 one layer 0.25 mm
Pure Lead Pure lead, 1/64th inch thick 0.4 mm
1. Characterize Spectra
Ten spectra were used, each using 10 mm of added Al filtration to harden the beam and approximate the spectrum of scattered radiation that would escape from a patient. The generator was calibrated to ensure accurate kV, and the half-value layer (HVL) of each spectrum was measured experimentally and compared with the theoretical value to confirm the spectral shape was well characterized. The following table describes the spectra used.
kV Added Al (mm) Theoretical HVL
(mm Al)
Measured HVL
(mm Al)
60 10 4.7 4.8
70 10 5.5 5.6
80 10 6.2 6.2
90 10 6.9 6.9
100 10 7.5 7.4
110 10 8.0 7.9
120 10 8.5 8.3
130 10 8.9 9.0
140 10 9.3 9.5
150 10 9.7 9.9
2. Measure Protection Factor
The protection factor of each sample for each spectrum was measured using an air ionization chamber and Keithley electrometer (Model 35617). Narrow-beam geometry was used to prevent contamination from scatter. Results are summarized in the following
table.
kV mA nCoul Protection Factor
No Sample Sample Pure Sample Sample Pure
Sample #1 #2 Lead #1 #2 Lead
60 250 3.808 0.033 0.162 0.050 115 23.5 76.2
70 50 1.427 0.026 0.099 0.045 55.0 14.4 31.7
80 50 2.834 0.030 0.271 0.145 25.6 8.8 16.4
90 50 3.588 0.230 0.573 0.334 15.6 6.3 10.7
100 50 5.01 0.389 0.922 0.550 12.9 5.4 9.1
120 50 8.48 0.743 1.743 1.019 11.4 4.9 8.3
130 80 0.332 0.0279 0.0681 0.0402 11.9 4.9 8.2
140 80 0.385 0.0340 0.0720 0.0470 11.3 5.3 8.2
150 80 0.437 0.0428 0.0938 0.0543 10.2 4.7 8.0
3. Determine Lead-Equivalent Thickness
The protection factor for a range of lead thicknesses between 0 and 1 mm was calculated theoretically for each spectrum. Results are shown in Fig 2.
1 10 100 1000
0
0.1
0.2
0.3
0.4
0.5
0.6
0.7
0.8
0.9
1
Equiv
Pb
Thickness
(mm)
50 kV
60
70
80
90
100
120
140
150
Protection Factor
Figure 2. Calculated protection factor associated with different lead thicknesses for each spectrum. This data is used to determine the lead equivalent thickness of measured protection factors.
Figure 2 is used to determine the lead-equivalent thickness corresponding to measured protection factors for each sample and spectrum. Results in Figure 2 apply to any material as long as the protection factor is measured using the spectra as stated in this report.
4. Scatter
All measurements described in this report were performed using narrow-beam geometry to avoid contamination from scatter radiation. As a result, negligible scatter is generated within the protective devices. In practice, a small amount of scatter can be generated within both lead and non-lead protective devices. Radiation “build-up” was measured in all three samples. A build-up of up to 15% was observed in both the lead and non-lead materials, confirming that the non-lead material is equivalent to the pure lead sample.
RESULTS
The calculated lead equivalence for each sample is summarized below and in Fig. 3.
kV Measured Lead-Equivalent Thickness (mm)
Sample #1 Sample #2 Pure Lead (0.40 mm)
60 0.47 0.27 0.40
70 0.50 0.30 0.42
80 0.50 0.29 0.40
90 0.49 0.29 0.40
100 0.49 0.29 0.40
120 0.49 0.28 0.39
140 0.49 0.31 0.41
150 0.48 0.29 0.42
The accuracy of the calculated lead-equivalent thickness is estimated to be 0.02 mm of lead. The equivalent thickness of the pure lead sample agreed with the known true thickness within this uncertainty. This agreement confirms that the analysis was
conducted accurately.
Sample #1 was found to have a 0.5 mm lead-equivalent thickness within the accuracy of the measurement over the energy range tested.
Sample #2 was found to have a 0.25 mm lead-equivalent thickness within the accuracy of the measurement over the energy range tested.
60 70 80 90 100 110 120 130 140 150
0
0.1
0.2
0.3
0.4
0.5
0.6
0.7
0.8
0.9
1
Pb-Equivalent
Thickness
(mm)
a) 0.40 mm Pure Pb
b) Sample #1
c) Sample #2
60 70 80 90 100 110 120 130 140 150
0
0.1
0.2
0.3
0.4
0.5
0.6
0.7
0.8
0.9
1
Pb-Equivalent
Thickness
(mm)
a) 0.40 mm Pure Pb
b) Sample #1
c) Sample #2
kV
Figure 3. Summary of measured lead-equivalent thicknesses for: a) 0.40 mm pure Pb; b) Sample #1
(nominal 0.5 mm); and c) Sample #2 (nominal 0.3 mm). Results are accurate to approximately 0.02 mm of lead.
We’re Giving Ourselves Cancer
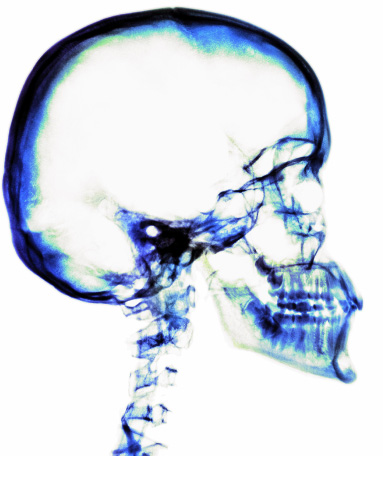
DESPITE great strides in prevention and treatment, cancer rates remain stubbornly high and may soon surpass heart disease as the leading cause of death in the United States. Increasingly, we and many other experts believe that an important culprit may be our own medical practices: We are silently irradiating ourselves to death.
The use of medical imaging with high-dose radiation — CT scans in particular — has soared in the last 20 years. Our resulting exposure to medical radiation has increased more than sixfold between the 1980s and 2006, according to the National Council on Radiation Protection & Measurements. The radiation doses of CT scans (a series of X-ray images from multiple angles) are 100 to 1,000 times higher than conventional X-rays.
Of course, early diagnosis thanks to medical imaging can be lifesaving. But there is distressingly little evidence of better health outcomes associated with the current high rate of scans. There is, however, evidence of its harms.
The relationship between radiation and the development of cancer is well understood: A single CT scan exposes a patient to the amount of radiation that epidemiologic evidence shows can be cancer-causing. The risks have been demonstrated directly in two large clinical studies in Britain and Australia. In the British study, children exposed to multiple CT scans were found to be three times more likely to develop leukemia and brain cancer. In a 2011 report sponsored by Susan G. Komen, the Institute of Medicine concluded that radiation from medical imaging, and hormone therapy, the use of which has substantially declined in the last decade, were the leading environmental causes of breast cancer, and advised that women reduce their exposure to unnecessary CT scans.
CTs, once rare, are now routine. One in 10 Americans undergo a CT scan every year, and many of them get more than one. This growth is a result of multiple factors, including a desire for early diagnoses, higher quality imaging technology, direct-to-consumer advertising and the financial interests of doctors and imaging centers. CT scanners cost millions of dollars; having made that investment, purchasers are strongly incentivized to use them.
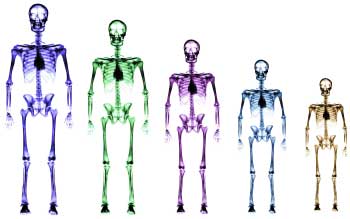
While it is difficult to know how many cancers will result from medical imaging, a 2009 study from the National Cancer Institute estimates that CT scans conducted in 2007 will cause a projected 29,000 excess cancer cases and 14,500 excess deaths over the lifetime of those exposed. Given the many scans performed over the last several years, a reasonable estimate of excess lifetime cancers would be in the hundreds of thousands. According to our calculations, unless we change our current practices, 3 percent to 5 percent of all future cancers may result from exposure to medical imaging.
We know that these tests are overused. But even when they are appropriately used, they are not always done in the safest ways possible. The rule is that doses for medical imaging should be as low as reasonably achievable. But there are no specific guidelines for what these doses are, and thus there is considerable variation within and between institutions. The dose at one hospital can be as much as 50 times stronger than at another.
A recent study at one New York hospital found that nearly a third of its patients undergoing multiple cardiac imaging tests were getting a cumulative effective dose of more than 100 millisieverts of radiation — equivalent to 5,000 chest X-rays. And last year, a survey of nuclear cardiologists found that only 7 percent of stress tests were done using a “stress first” protocol (examining an image of the heart after exercise before deciding whether it was necessary to take one of it at rest), which can decrease radiation exposure by up to 75 percent.
In recent years, the medical profession has made some progress on these issues. The American College of Radiology and the American College of Cardiology have issued “appropriateness criteria” to help doctors consider the risks and benefits before ordering a test. And the insurance industry has started using radiology benefit managers, who investigate whether an imaging test is necessary before authorizing payment for it. Some studies have shown that the use of medical imaging has begun to slow.
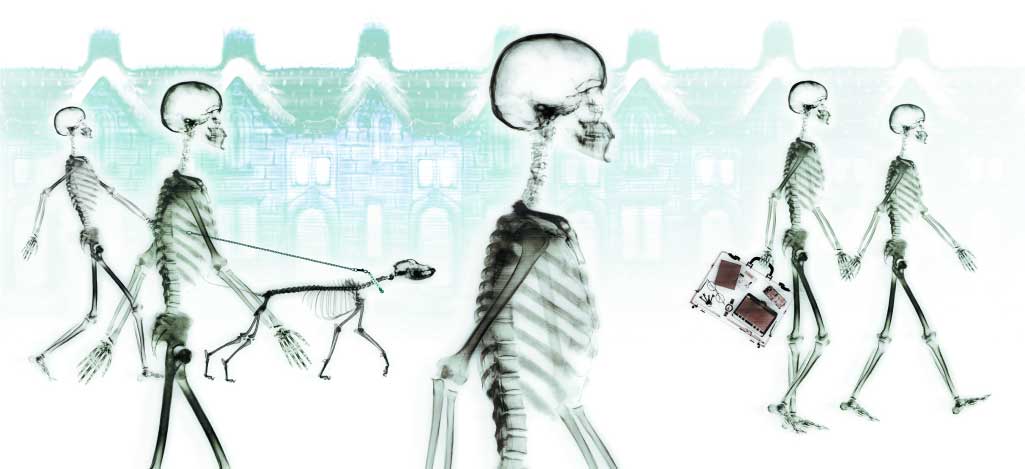
But we still have a long way to go. Fortunately, we can reduce the rate of medical imaging by simply avoiding unnecessary scans and minimizing the radiation from appropriate ones. For example, emergency room physicians routinely order multiple CT scans even before meeting a patient. Such practices, for which there is little or no evidence of benefit, should be eliminated.
Better monitoring and guidelines would also help. The Food and Drug Administration oversees the approval of scanners, but does not have regulatory oversight for how they are used. We need clear standards, published by professional radiology societies or organizations like the Joint Commission or the F.D.A. In order to be accredited for CT scans, hospitals and imaging clinics should be required to track the doses they use and ensure that they are truly as low as possible by comparing them to published guidelines.
Patients have a part to play as well. Consumers can go to the Choosing Wisely website to learn about the most commonly overused tests. Before agreeing to a CT scan, they should ask: Will it lead to a better treatment and outcome? Would they get that therapy without the test? Are there alternatives that don’t involve radiation, like ultrasound or M.R.I.? When a CT scan is necessary, how can radiation exposure be minimized?
Neither doctors nor patients want to return to the days before CT scans. But we need to find ways to use them without killing people in the process.
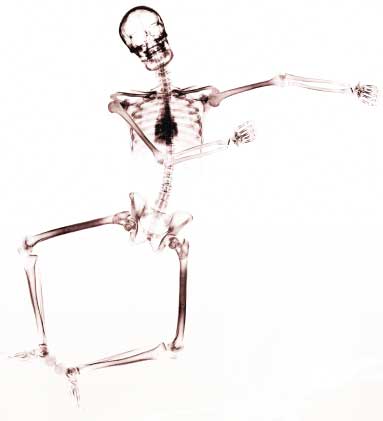
Univest-X has a role to play against radiation.
Univest-X provides quality products made entirely in Quebec
and ensures maximum protection in accordance with the highest standards set by Health Canada.